The evolution of the human species is the outcome of a long sequence of random processes, but that does not imply that we evolved by chance
Did the human species evolve by chance?
The answer, in one word, is “yes”, and in three words, “yes and no”.
The evolution of the human species, like that of any other species, is inevitably linked to random events. In each generation, individuals who are better adapted to their environment - those that manage to acquire food, escape predators, survive diseases and parasites and attract successful partners - are the ones to reproduce and pass on their traits to the next generation. This is the basic principle of natural selection, which works only when the variation within the population is high enough. If certain individuals manage to produce more offspring than others, due to specific traits, natural selection occurs.
In his book, On The Origin of Species, Charles Darwin, who formulated the Theory of Evolution by Natural Selection, referred to this variation as being caused by chance, although he was quick to clarify that the expression was only meant to convey the fact the causes for the variation were still unknown. Today, we know much more about these causes, the first of which is mutations - changes in the DNA sequence. Mutations can form due to a myriad of reasons, from exposure to radiation or certain chemicals to errors occurring during the replication of genetic material. In the majority of cases, mutations appear to occur randomly, although some studies show that under certain circumstances the rate of mutation can be different in different parts of the genome.
Random mutations are the main sources for the variation necessary for evolution to occur. However, this does not mean that evolution itself is a random process. The main factor that directs evolution is natural selection, which as its name suggests, selects the traits that help individuals survive and produce offspring, thus also selecting the mutations responsible for these traits. It is no coincidence that mammals living in the far north developed thick fur, or that insects living among tree branches have a shape similar to a stick or a leaf. These traits evolved because they were the most successful in the respective environments of these animals. However, each genetic change that contributed to these traits originally appeared by chance in one individual in the population and spread through the population by helping that individual reproduce. Evolution is driven by chance and necessity, as described in the book, Chance and Necessity, by the French Nobel Prize Laureate, biologist Jacques Monod.
It is worth noting that the “necessity” part, that is, natural selection, is also influenced by random processes, and it is essentially probabilistic. For example, white fur provides an advantage to a hare living in snowy areas, giving the hare a greater chance to escape predators, but it cannot guarantee that the hare’s will evade the teeth of a fox or a wolf, and it certainly cannot protect the hare from disease or ensure that it will find a mate. We can predict that over time, more white hares will survive and produce offspring than those with brown summer fur in the winter, and thus the genes for white fur are more likely to spread through the population. But we cannot predict with certainty the fate of each and every individual in the hare population. These are just some of the probabilistic processes involved in evolution. In this article we will explore how chance has affected and continues to affect the evolution of living organisms, including humans, and why, nevertheless, we did not “evolve by chance”.
Natural selection is also affected by random processes, and is essentially probabilistic. A drawing of Darwin next to dice cubes. Image | Yonat Eshchar using Midjourney
What Do Mutations Do? Mostly, Nothing
Our genetic material, DNA, is found in every cell of our body and is largely responsible for determining our traits. This is not done directly: the DNA is like a set of instructions according to which our cells’ proteins, which are the main executors of the different processes in our body, are constructed. Mutations, or changes in DNA, can alter the structure and/or function of a protein, such that it either fulfills its role differently, for example faster or slower, or is ineffective in fulfilling its function. In rare cases, a mutation can cause a protein to perform something that it did not perform beforehand. Furthermore, mutations in other regions of the DNA sequence may also affect the amount of protein produced or change the location of its production in the body.
While mutations occur constantly in the cells of our body, the mutations that are most most important for the process of evolution are the ones that occur in reproductive cells (sperm and egg), since these mutations are the ones to be passed on to the next generation. Studies have shown that every child has about ten to twenty new mutations, which were not present in their parents.
Does this mean that the child will exhibit new traits that were not present in their parents? There’s no certain answer for this question.The absolute majority of changes in the genetic material do not lead to any alterations in proteins. This is because a considerable portion of our DNA does not contain genes, which are the sequences that provide instructions for the construction of proteins. So what does it contain? Some of it consists of DNA sequences that are crucial for controlling protein synthesis, but most of it, at least as far as we know to date, does not serve any purpose. This includes genes that are no longer functional, viruses that entered our genome many generations ago, and more. Mutations in such sequences will have no effect whatsoever on proteins.
Even mutations within the protein-coding genes, which serve as the instructions for protein synthesis, may not necessarily result in changes in proteins, but may cause small changes in sequence that will not be reflected in protein activity. Such mutations are called “silent mutations”, and most researchers agree that they do not affect the living organism in which they occur, although others claim that they have a harmful effect.
A study in bacteria revealed that over 98.5% of the mutations are neutral, and have no effect on the functioning of the bacterium. For evolution, the important mutations are of course those that affect the traits of the living organism. Let’s focus on those.
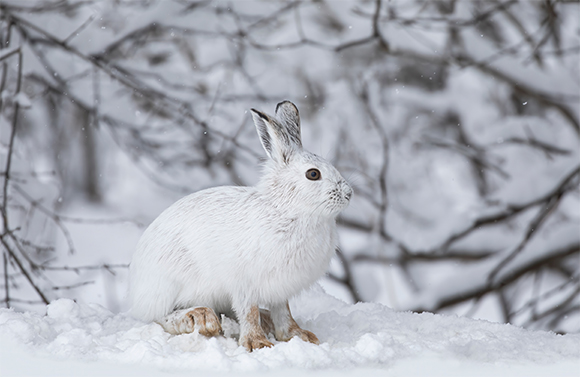
White fur will provide an advantage to an animal that lives in snowy areas. A white Snowshoe Hare in a snowy environment | Jim Cumming, Shutterstock
What Do Effective Mutations Do? Mostly, Harm
Among the mutations that aren’t neutral, nearly all are deleterious. It’s easy to see why this is so if we compare a gene that contains instructions for the construction of a protein to a cake recipe. Let’s assume that the recipe contains the line “one and a half cups of sugar”. A “mutation” in the recipe may change this to “one and a half cups of vinegar” or to “one and a half cups of lettuce”. Both options would result in a cake that no-one would want to eat. Since mutations are random, they could even lead to instructions such as “one and a half cups of vacation” or “a cat and a half cups of sugar”, which have no meaning and any attempt to implement them would probably result in us not obtaining a cake at all.
Is there a mutation that could improve the cake? This is definitely likely: the line “one and a quarter cups of sugar”, for example, may result in a cake with more balanced flavors, which would possibly be tastier. However, while the possibilities for destroying the cake, as we have seen, are countless, there are far fewer ways to improve it.
The same thing takes place in our genes. Proteins are sophisticated, delicate machines, and if a mutation in a gene leads to a change in the protein, most chances are that it will impair its function. Therefore, the main role of natural selection is to filter out the harmful mutations from our genome: if a mutation causes an animal to have greater difficulty surviving or reproducing, there is little chance that it will be passed on to the next generation. However, the process of filtering out the harmful mutations is not always efficient. Some of the mutations are recessive, that is, they become manifested only if we have two copies of them: one in the gene we receive from our father, and one in the gene that comes from our mother. If we carry such a mutation in only one of the genes, but not in both, we will not even be aware of it, and we will pass it on to our offspring. This is one of the ways by which deleterious mutations, such as specific mutations that cause severe genetic diseases, nevertheless remain in the population for many generations.
Most of the mutations that are not neutral are harmful. A mutation in DNA | Source: andriano.cz, Shutterstock
Beneficial Mutations Spread through the Population - Eventually
What happens when a mutation, which is not harmful but instead advantageous, occurs in a particular population? Let’s consider the case of brown American black bears. Yes, you read it correctly - these are American black bears with brown fur. Typically, American black bears (Ursus americanus) are usually black, hence their name. However, around 9,000 years ago, in what is now western United States, a single mutation occurred in a particular gene, causing the color of its owner’s fur to be ginger-brown, also known as “cinnamon”, as researchers call it. It seems that the new coloration provides a camouflaging advantage to the bears, enabling them to blend into their forested surroundings, protecting their cubs from predators such as pumas and wolves, and allowing the adults to approach their prey without being detected.
If this mutation is beneficial, why hasn’t the “cinnamon” coloration become dominant in the American black bear population, even after the thousands of thousands of years and hundreds of generations that followed the appearance of the mutation? Why hasn’t natural selection caused this mutation to spread throughout the population?
This brings us back to the issue of probability. Even when a beneficial mutation appears, there’s no certainty that it will spread throughout the population, let alone quickly. Despite the brown fur that helps the cubs to blend in, there’s still a chance that the first individual carrying this mutation may fall prey for a wolf, drown in a flood, or succumb to a disease. In such cases, the mutation will disappear without leaving a trace, and the population will remain black until another mutation with a similar effect appears.
Even if the carrier of the mutation manages to survive and reproduce, there is no guarantee that the mutation will be inherited by the offspring. Let’s say the first brown bear had three cubs. What are the chances that the mutation will be passed on to them? Each sperm cell contains half of the bear’s genetic material, that is, only one copy of each gene, instead of the two copies found in any other type of cell. Thus, the probability of a mutation in a single gene being inherited by the offspring is one in two, or fifty percent. With respect to the three cubs, the chances increase - now there is a seven in eight chance that at least one of the cubs will inherit the mutation, and only a one in eight chance that it will not be passed on to any of them. However, a one in eight chance, or 12.8%, is not that small. In other words, there is a very reasonable chance that the bear with the beneficial mutation, even if it survives and produces offspring, may die without passing the mutation on to the next generation.
In the case of the “cinnamon” mutation, it was indeed passed on to subsequent generations, and spread through the population, and it seems that it is continuing to spread—but it is doing so slowly, because the advantage it provides is not particularly high and merely probabilistic. “Classic” American black bears can still survive and reproduce, thus their genes also continue to be passed on. The distribution of the “cinnamon” gene also depends on the region in which the brown bears reside and with whom they mate. The mutation appeared in the west, and even now, thousands of years later, most of the brown bears can be found in that region. Emily Puckett, who led the research, said in an interview for the Live Science website, that it is not easy for the cinnamon gene to reach the bears living in the Eastern United States. According to her, the bears don’t pass through the Great Plains found in the center of the country, since the environment there is not suitable for them. “If they wanted to go east, they would have to go up north to Canada, across the Canadian Prairies, around the Great Lakes and then drop back down into the eastern populations”, says Puckett. The fact that the same mutation has been observed in the east indicates that there were individuals that have indeed migrated and at least one of them was a carrier of the cinnamon mutation. But this is another stage in which random factors affect the spread of the gene: factors such as which bears choose to migrate, which ones survive the journey, and which bears they mate with. These factors will determine when the gene reaches the eastern population and how fast it will spread throughout it.
Why doesn’t a beneficial mutation always reach every individual in a population? Because of the laws of probability. A cinnamon-colored American black bear | Michael J Magee, Shutterstock
Drifting with Genetics
The case of the brown American black bears illustrates how probability and chance play a significant role in the fate of a particular gene - even in a very simple case, in which one mutation affects one essential trait of the bears - the color of their fur. However, in more complex cases, multiple mutations in various genes have a joint impact on the same trait, or one gene may affect several traits. In other cases, different mutations can either cancel out or amplify each other’s impacts.
When dealing with multiple mutations, the hereditary process becomes even more complex. Our genetic material is packaged into 23 pairs of chromosomes - long chains of DNA. A neutral mutation that occurs in close proximity to a beneficial mutation on the same DNA chain may spread throughout the population solely by virtue of its location; essentially “hitchhiking” on the beneficial mutation that increases the chances of its owner to survive and reproduce.
However, the chromosomes are not passed down unchanged from parents to offspring. During the production of sex cells - sperm and egg cells - chromosomes undergo a process of recombination, in which they exchange fragments of DNA with their counterparts. For exsmple, chromosome 1 from the father will exchange fragments with the same chromosome 1 from the mother, chromosome 2 with its conuterpart and so on for all chromosomes. This recombination process results in a mixing of genetic material from different ancestries. It can also separate mutations that are located close to each other and create new combinations that did not exist previously.
Genetic drift is particularly evident in small populations. To illustrate why, let’s consider the following scenario. Suppose we have a population of dogs, half of which have black fur, and half white fur. The color of the fur, in this case, doesn’t affect their ability to survive, find food or reproduce. However, only one quarter of the dogs in each generation manage to produce offspring and pass on their traits, including fur color, to the next generation.
In a population of one million dogs, about 250,000 will reproduce and it is unlikely that all of them will have the same fur color - some will be white and some black. The chance that all of those dogs will have the same color is negligible. However, a population of only 40 dogs, only ten of which can reproduce, is still likely to include both black and white dogs ,the chance of all of them having the same fur color is much greater - still very low, but significantly higher compared to the previous example. And, of course, evolution acts over many generations.If seven out of ten dogs are black and only three are white, in the next generation the color ratio will not be 50-50, but approximately 70% black and 30% white. When this new generation reproduces, there will already be a greater chance of obtaining only black dogs. The gene variant for white fur color could disappear from the population, not due to being harmful, but simply owing to genetic drift: random processes that have a significant evolutionary impact on small populations.
An example of genetic drift: out of 40 dogs only ten will produce offspring, and over the generations the gene for white fur will disappear due to the small population size. 7 black dogs and 3 white dogs | Alemon cz, Shutterstock
Mutations of Survivors
This is of course a simplified example used only for illustration purposes. It does not take into account the complexity of dominant and recessive mutations and doesn’t consider a host of other factors that affect gene distribution in a population. But we can also find examples of genetic drift in the real world. Some of these refer to a phenomenon termed a “bottleneck”: when an animal population experiences a sudden decrease in numbers, a significant portin of its genetic diversity, including unique mutations found only in some individuals, can be lost. As the population begins to recover, the genes that dominate it will be those that were present, by chance, in the surviving individuals, while all the rest will no longer exist.
Consider the case of the Northern elephant seal (Mirounga angustirostris) as an example. In the late 19th century these marine mammals were hunted until only about 30 individuals remained. In the late 19th century, humans hunted these marine mammals almost to extinction, leaving only about 30 individuals alive. After receiving protection they began reproducing again, and currently their population numbers tens of thousands—but their genetic diversity remains very low. Many potentially beneficial mutations, such as those for a more optimal fin shape or improved immunity against viruses, disappeared along with the individuals who carried them. Only the genes that were present in the few surviving seals were passed down to subsequent generations. While new mutations will eventually appear with time, they will likely be different from those that were lost.
Human populations are also susceptible to bottlenecks. In 1775, a typhoon hit the island of Pingelap, found in Micronesia, in the Pacific Ocean, leaving few survivors from the originally small population. One of these survivors carried a rare mutation that causes achromatopsia, a condition of severe color blindness and increased sensitivity to light. While the island population has since recovered, about 10% of the island’s inhabitants still carry the mutation, almost 250 years after the typhoon. In contrast, this mutation is found in only 1 in 30,000 people in the general population. Thus a mutation that is not beneficial and may even be harmful, has become widespread in a specific population simply because it was present in one of the few individuals who survived the typhoon.
Owing to the bottleneck phenomenon, tens of thousands of individuals living today have a reduced genetic diversity. Northern elephant seal | Gerry Matthews, Shutterstock
The Song of the Founding Mynas
A similar phenomenon occurs when a small group of individuals from a large population colonize a new area, such as an island, or for marine animals, a sea that is separate from the large ocean. As these individuals begin to reproduce, the population that forms in the new location will carry the genes and mutations that were present, by chance, in the first individuals who reached that place. This phenomenon is known as the “founder effect”, and has a similar effect to a bottleneck, although its causes are distinct.In both cases, an entire population is established relatively quickly from a small number of individuals, leading to limited genetic diversity of the population.
The founder effect was observed, for example, in the common myna (Acridotheres tristis), a bird that many of us are familiar with. While originating from southern Asia, some mynas were brought to the Zapari Bird Sanctuary in Ramat Gan in the 1990s, escaped from their cage and spread throughout Israel. All of the mynas living in Israel today are likely descendants of those same few individuals from the Zapari, so even though Israel is not an island, this is still a case of the founder effect. In a study published in 2018, researchers compared populations of mynas in their natural distribution range to those in which they constitute an invasive species, such as Israel, coming from South Africa to Australia.They discovered that in the invasive populations the mynas had fewer songs, and that their songs were more similar to each other than those observed in Asian populations. The founder effect influenced the mynas’ songs just as it influenced their genes: since they are all offspring of a limited number of birds, with a limited number of songs, the diversity remained low even after the population grew.
An entire population formed relatively rapidly from a small number of individuals, leading to limited genetic diversity, this time due to the founder effect. The common myna | Only Fabrizio, Shutterstock
Replaying the Tape
In his book Wonderful Life, biologist Stephen Jay Gould posed an intriguing question - what would happen if we “replay the tape of life”?. If we were to turn back the clock 100 million years to the Age of Dinosaurs, or even 500 million years, to the time when multicellular life had just begun to emerge on Earth, and allowed evolution to unfold anew - from the very beginning - what kind of world would we end up with? Would we obtain the same world of flora and fauna that as the one we have today, or would everything be completely different?
Gould argued that the second possibility would be the correct answer to that question. Evolution is influenced by a range of random factors, including the mutations themselves, the inherent probability of the hereditary process as well as chance events that enable a specific individual to survive a natural disaster while others perish. These factors imply that in every “replay” of evolution, distinct individuals with a unique set of genes will survive and pass those same genes on to the next generation. Consequently, the evolution of living organisms will occur along a distinct pathway each time, resulting in markedly different outcomes.
This is of course, a thought experiment - evolution on Earth took place only once, and we cannot test what would happen if it were to occur again. However, we can conduct an experiment with several similar characteristics, on a much smaller scale. In 1988, American biologist, Richard Lenki, began such an experiment, with twelve colonies of E. coli bacteria. Initially, the colonies were completely identical, but bacteria reproduce rapidly, and with each cell division, mutations accumulated in the different colonies.The researchers monitored the mutations that developed in the bacteria over the years and found that some of them were beneficial. For example, some colonies acquired the ability to digest a new type of nutrient, while others did not. The researchers also periodically took samples from the colonies and froze them, so that the bacteria did not reproduce, but also did not die.
This experiment enabled the researchers to essentially “replay the tape” - not of all life, but at least of these bacteria. For instance, they dethawed a sample taken five years earlier from colony 3, cultured the bacteria from this sample for a period of five years, and examined whether they developed the same traits found in the bacteria of this colony in the first “run” of the experiment. The findings supported Gould’s argument: different mutations were discovered in each colony and even in samples taken from the colonies and grown at a later date, resulting in development of different traits. One colony developed the ability to exploit new food after around 30,000 generations, but its capacity to do so was affected by mutations that occurred thousands of generations earlier: colonies lacking those same seemingly neutral mutations never acquired the ability to to exploit this nutrient as an energy source. Gould would have claimed that these mutations set the colony on a trajectory that leads to an ability to exploit this nutrient, while other colonies followed a different course.
The experiment examined whether a new “run” of the same bacterial colonies leads to different mutations and traits. Colonies of the bacterium, E. coli | AnaLysiSStudiO, Shutterstock
Chance and Necessity
If we really could replay evolution, would humans be the inevitable outcome? And would they resemble us? While different researchers will answer this question differently, it seems that the general consensus is that the answer to the first question would be “not for certain” and to the second, “almost certainly not”.
Yes, there are certain traits that would be expected to evolve, sooner or later, in any “replay” of evolution. For instance, some of the sea-dwelling organisms would probably develop fin-like organs since they are highly efficient for movement in water. Similarly, animals that fly would have wings, which would probably resemble those of birds or bats, or those of flies or dragonflies, depending on the animals’ size. There aren’t many other effective ways to move through the air. Natural selection drives living organisms to evolve traits and behaviors that help them survive and reproduce in their environment, and sometimes very different organisms evolutionarily arrive at similar “solutions” - a phenomenon known as convergent evolution. However, as we’ve observed, evolution is also influenced by many random factors that can also impact those same traits.
Our history shows that a large brain and quick hands provide a significant advantage in certain environments, and that a species carrying such traits can thrive and reproduce.It’s quite possible, therefore, that animals similar to humans in some respects would almost inevitably evolve at some point during the course of evolution. However, this does not necessarily imply that they would be exact replicas of humans, with the same number of fingers and toes and limbs, or with a similar lifestyle.Rather, these characteristics would be shaped by the evolutionary path taken by those hypothetical animals, and the various events that did or did not take place along that path.
Did humans evolve by chance? The answer is both “yes” and “no”. Our unique evolutionary history is shaped by millions and hundreds of millions of random events. However, there is a reason that a social animal, with a large brain, language and dexterous hands gained a survival advantage, reproduced and passed its traits onwards. It just so happened that, by chance, that animal was us.